Cell talk in the growth of blood vessel grafts
Jordy van Asten looked at ways to model how cells communicate with each other in grafts for blood vessels for his PhD research, and now he’s off to explore the communication of science with the world.
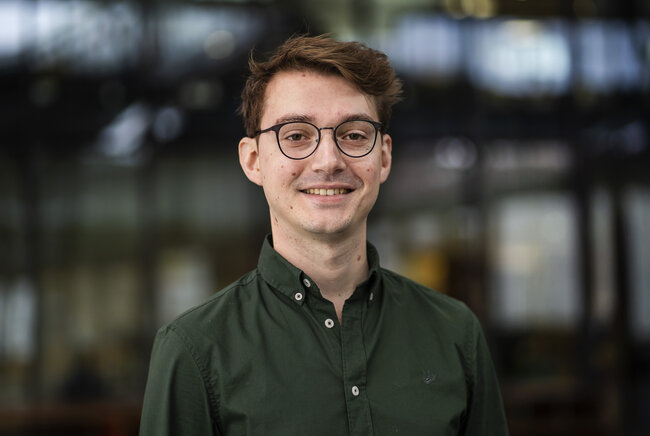
Patients suffering from cardiovascular diseases can need new blood vessels in the form of grafts to replace or bypass diseased vessels. Current graft options include using vessels from other parts of the body or fully synthetic vessels, but these options have limitations. Recent advances in tissue engineering have seen the emergence of vessels made from biodegradable materials, and once placed inside the body, the cells needed to make vascular tissue are attracted to live in the vessel. Key to this process is communication between cells in the engineered vessels, and this is still poorly understood. So, PhD researcher Jordy van Asten turned to computer models to help in understanding communication between cells.
A combination of curiosity and impact. That’s the reason why Jordy van Asten embarked on a research adventure in the field of tissue engineering, which he concluded on June 7th when he defended his PhD thesis at the Department of Biomedical Engineering.
“I’m an engineer, and for me, the human body is the most fascinating ‘machine’ to investigate,” Van Asten says with enthusiasm. “It’s amazing to see how it works and how intricate and clever it is. So, I’ve always been curious to learn about it and try to understand it.”
As a machine, the human body consists of many parts working together. Critical to all these parts is the supply of blood carrying oxygen, water, nutrients, and other key molecules. “Vessels form part of the blood-carrying network. When someone suffers from a vascular disease, it’s often the case that blood vessels are damaged and need to be replaced with grafts,” says van Asten.
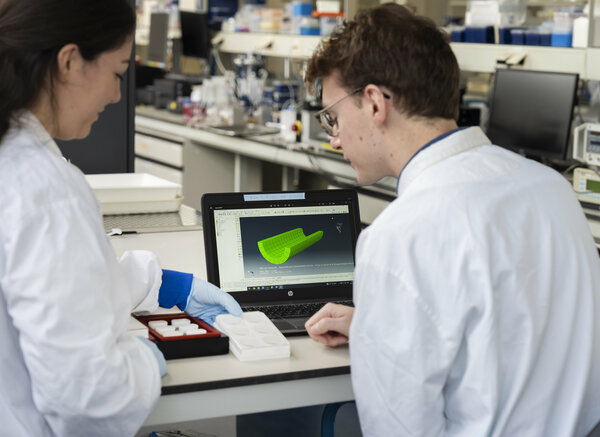
Meet the tissue engineering option
So, what are the current replacement options for vessels then? van Asten: “There are two current graft options. First there’s autologous vessels, which are vessels taken from another part of the patient’s body. And second there’s the option to use a synthetic graft that’s made from polymer-based materials, for example.”
These options have some serious limitations though. Suitable autologous vessels are limited, and they may not work well in the long-term. Synthetic grafts have numerous problems. They narrow a lot when used to replace small-diameter vessels, and they cannot grow with the body. The last part is a worry when implanted in young patients who eventually outgrow the graft and it needs to be replaced once again with surgery.
“And that’s where tissue engineering may come to the rescue, which involves making a replacement blood vessel in the laboratory that can be placed inside the body,” says van Asten. “Over time, the vessel can grow and adapt with the body.”
Key to tissue-engineered vessels is the use of degradable biomaterials to make a scaffold. “The scaffold can be made into the shape of the blood vessel that’s needed,” notes van Asten. “Once it’s placed inside the body, we exploit the inflammatory response of the body to send cells to the scaffold and guide tissue formation. The cells grow and make new vessel tissue in the scaffold. And because the scaffold is biodegradable, it eventually dissolves inside the body leaving behind a new working blood vessel in its place.”
Cells calling
To date, there have been some very promising results. However, the long-term functionality and reproducibility of tissue-engineered vessels still require improvement before this technique can be used in patient treatments.
“Understanding and controlling the growth and remodeling of blood vessels are the big things,” van Asten highlights. Here, growth is the change in vessel volume, while remodeling is the change in material structure and properties.
“Cells drive growth and remodeling, and cell communication is integral to this, such as Notch signaling, which influences how cells behave,” notes van Asten. “And to do this, I turned to computer models.”
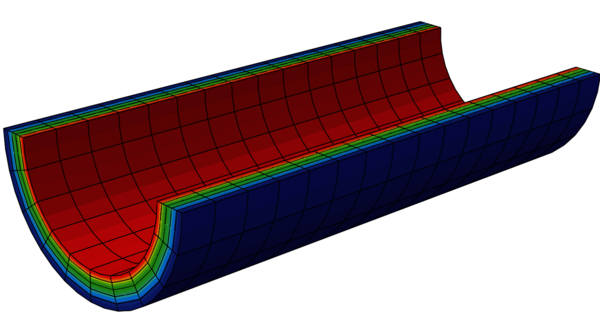
Models calling
van Asten and his colleagues developed computer models to simulate how blood vessels grow and are remodeled in two different cases.
“In the first case, we wanted to explore the thickening of native vessels due to an increase in blood pressure and use this as a way to monitor growth and remodeling together,” says van Asten.
Pressure stretches cells in the vessels, and this affects how strongly cells communicate via Notch signaling. Simulation results showed that Notch signaling played a key role in the thickening of blood vessels under increased pressure. “When the cells were stretched, Notch signaling between cells was affected such that more cells grew,” says van Asten. “The results also show that we can change vessel geometry and composition by manipulating Notch signaling.”
In the second case, van Asten simulated the formation of tissue-engineered blood vessels from scaffolds under different conditions that might be found inside the body. “Our model shows that these conditions can lead to variability in the composition and functionality of the tissue grown by affecting growth and remodeling.”
He also looked at simulations where certain proteins were added to the surface of the scaffold to change how Notch signaling worked between cells. “The proteins altered growth and remodeling in the vessels, but the variability was the same. Some vessel properties were better, while other vessel properties were worse.”
Science communication calling
Despite the promising results, van Asten is quick to highlight that Notch signaling is just part of the story. “This is not the only mechanism determining vessel growth. The models include several other mechanisms, but my focus here was on Notch signaling only. For the future, more experimental data is needed to validate the models and other ways that cells communicate with each other should be considered too. This type of model could have exciting consequences for improving tissue engineering.”
Significant advancements are expected in the field of tissue engineering then, but van Asten won’t be part of the future revolution in that field as he is changing course in his career journey as he has changed to the world of science communication.
What attracted van Asten to science communication? “I love the challenge of translating complex scientific topics for different audiences. It requires you to think very carefully about your main message: ‘what should people really know and understand?’”
van Asten has been working as a communication advisor at Utrecht University since February this year, and he’s enjoying the change so far. “I’m contributing to the impact of research, and really enjoying it. Science should not be done only for science’s sake. I believe it should somehow contribute to our society and a better world. It’s a lot of fun to learn about fascinating research, translate that research into digestible messages for other audiences, while hopefully inspiring people in the process.”
Title of PhD thesis: Modeling mechano-regulated Notch signaling in arterial growth and remodeling. Supervisors: Sandra Loerakker, Cecilia Sahlgren, and Tommaso Ristori.
Media contact
Latest news
![[Translate to English:] [Translate to English:]](https://assets.w3.tue.nl/w/fileadmin/_processed_/6/2/csm_universiteit%20jos%20keurentjes%20energie%202_d469fe3b2f.jpg)
![[Translate to English:] [Translate to English:]](https://assets.w3.tue.nl/w/fileadmin/_processed_/2/2/csm_Pre-EditLeviBaruch-0003_558369f155.jpg)
![[Translate to English:] [Translate to English:]](https://assets.w3.tue.nl/w/fileadmin/_processed_/7/d/csm_Boutin%20Banner%20image_165e239d2b.jpg)